Yvonne Shashoua
National Museum of Denmark
Malignant! Wicked! Toxic! These terms might bring to mind descriptions of some world leaders or conflict situations, but in actuality they have all been recently used to describe plastics in our material heritage and archaeological sites (Scott 2002 and British Archaeology 2024). While I might use the slang âwickedâ to describe the plastic spacesuit worn by Andreas Mogensen, the first Danish astronaut in 2015, I cannot imagine that professional bodies like ICOM and EAA applaud the application of such negatively biased terminology to the materials in our care. In our modern world, where the policies and practices of inclusivity are promoted, I question why plastics are being cancelled in archaeology publications and how it affects their perceived value as artefacts as well as for use in both conservation and as storage materials.
First, I will introduce plastics and why they degrade from a scientific perspective. Next, I follow with three cases of heritage materials to illustrate why archaeology should not cancel plastics. I contend that plastics are both a substantial part of our material heritage in modern times and an important tool in contemporary archaeological work.
Plastics 101
Plastics comprise macromolecules or polymers (more than 99% by weight) that are modified with additives (antioxidants, thermal stabilizers) and shaped to convert them from liquids to solids. Polymerisation reactions, the selection of additives and fillers, and shaping technique all contribute to the physical and chemical properties of plastics, and to their degradation pathways and lifetimes.
The polymer component in the first manmade plastics (developed between 1850 and 1910), originated from natural sources such as cellulose from trees and casein protein from milk. Natural polymers were chemically treated with acids (cellulose acetate and nitrate) or formaldehyde (casein-formaldehyde) to modify their chemical properties and produce physically stable, mouldable products. However, since the 1950s, almost all plastics have been synthetic, and their polymer components formed by chemically bonding together thousands of small gas molecules such as ethylene, propylene and styrene (monomers) rather than chemically treating natural polymers. Because of their low cost, 96% of monomers are derived from crude oil, natural gas or coal, all of which are non-renewable or fossil sources (Plastics Europe 2021). Although it is possible to synthesize chemically identical polymers from renewable resources such as waste biomass, less than 5% of plastics produced today are bio-based. This discrepancy is largely due to cost.
The degradation of plastics may be envisioned as a reversal of their chemical syntheses. When plastics are exposed to oxygen in air, heat, light or mechanical forces, the additives that protect polymers work until they are exhausted. As exposure continues, the chemical bonds comprising the side chains and backbone of the polymer break sequentially from the weakest to the strongest. Light, particularly ultraviolet (UV) light with wavelengths 200 - 800nm, is the most damaging factor leading to the degradation of plastics. Because bond energies in polymers are typically between 300 and 500kJ per mole, they can withstand visible and infrared light with energies 300 and 180kJ per mol respectively. By contrast, UV light contains sufficient energy to break the strongest chemical bonds in polymers (McNeill 1992). This results in physical disintegration of plastics.
In heritage collections, significant symptoms of plastic degradation include yellowing, stickiness, shrinkage, brittleness and flaking. Together, these result in loss of function, form and/or interpretation. In natural environments (including archaeological sites), the most frequently recorded symptom of plasticsâ degradation is their fragmentation into particles or fibers known as microplastics (<5 mm) (Zhang et al. 2021). Identified for the first time in 2004, microplastics are the largest particles that can be inhaled or ingested and subsequently transported into the living tissues of animals, fish, birds, insects, and humans (Thompson et al. 2004). Microplastics are not the final stage of the degradation process. In fact, they merely represent the last stage detectable by the naked eye. Smaller nanoplastics (0.05-5mm) have been detected in air, water, and food (Chen et al. 2021) and the complete degradation of plastics to carbon dioxide in soil is known as mineralization (Huo et al. 2024).
The first carbon-neutral, bio-based plastic dates from 1862
Although bio-based plastics are thought of as novel materials, the very first plant-based plastics date from 1862 when they were made by reacting natural cellulose derived from cotton and fibres or wood pulp with concentrated acids (Stannett 1951). One advantage of this from an environmental perspective is that plants store atmospheric carbon dioxide (CO2) during growth. Therefore, using bio-based sources to produce plastics removes CO2 from the atmosphere and fixes it throughout a productâs lifetime (Weiss et al. 2012).
Causing cellulose to react with acids (known as acetylation), transforms the insoluble, non-formable, cellulose polymer into readily soluble, transparent cellulose acetate plastic that can be cast into sheets and spun into textile fibers. In the early 20th century, cellulose acetate replaced rare and expensive natural materials such as ivory, tortoiseshell, and mother of pearl in high value, functional products such as hair combs, spectacle frames and cutlery handles (Meade et al. 1905). It was also substituted for inflexible, heavy glass in photographs and tracing sheets.
Because acetic acid is the major product of deacetylation, and the degradation of artefacts and photographic film gives off a vinegar-like odour, the process is also known as âvinegar syndromeâ. Importantly, acetic acid vapour also catalyses degradation of other organic materials, including paper, and it corrodes metals. See Figure 17. If unchecked, 5-20 years after the initial symptoms of deacetylation are visible, cellulose acetate is reduced back to natural cellulose. In contrast to synthetic polymers based on fossil sources (that degrade to microplastics), cellulose acetate forms paper fibres that are considered harmless to human and animal health. See Figure 18.
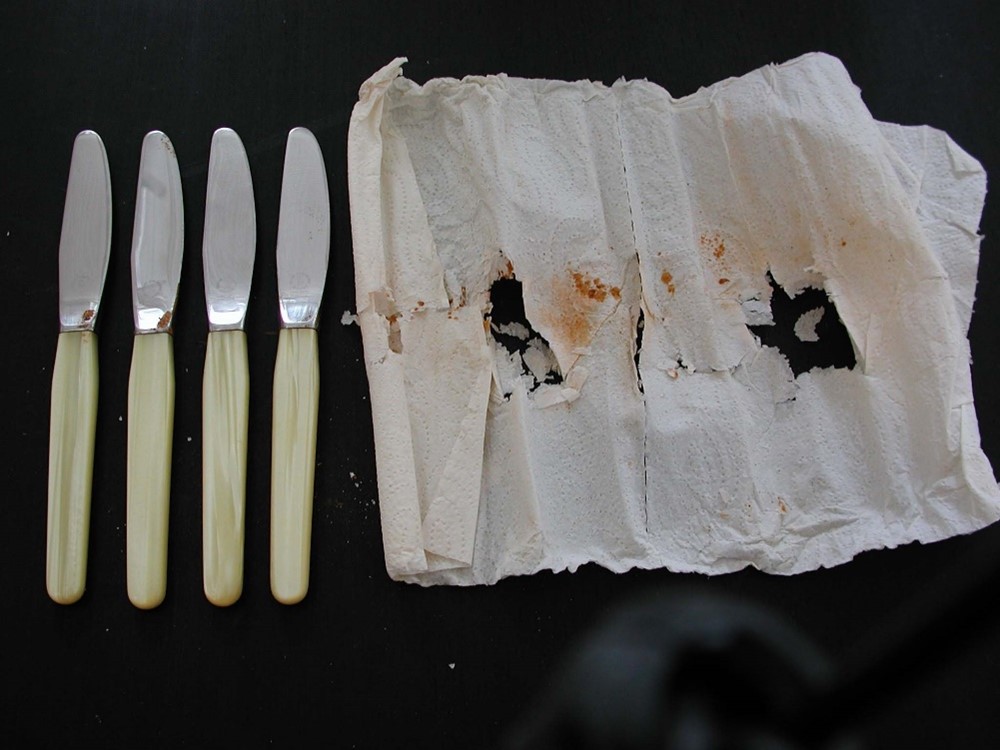
Figure 17. Acetic acid produced by degrading knife handles from the 1950s has attacked the tissue in which they have been wrapped. The knife blades have been corroded by acetic acid.
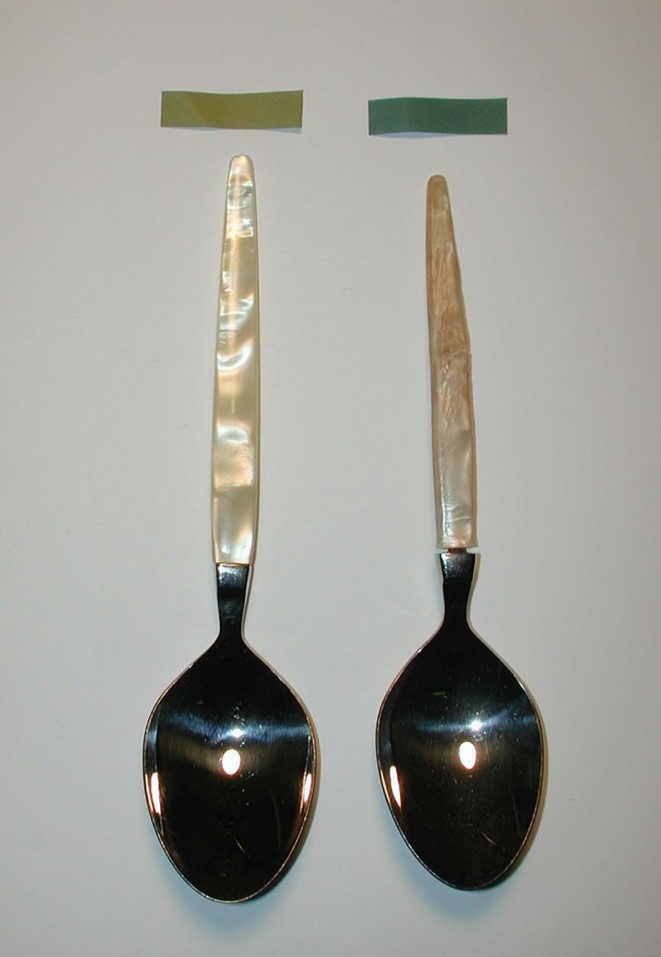
Figure 18. Acid detection strips enclosed with the spoons indicate that the spoon handle in good condition (left side of image) gives off acetic acid, while the highly deteriorated example (right side of image) produces none. The handle on the right has degraded to cellulose.
No plastics - no space suits
Although its first application was as an effective replacement for rubber in electrical cable insulation during World War II, today plasticized polyvinyl chloride (PVC) is the second most highly consumed plastics material in the world. This is mainly due to its high flexibility (think of, e.g. garden hoses), its excellent ability to serve as a barrier to air and water (e.g. blood and colostomy bags, inflatable furniture, shower curtains), high flame retardancy (e.g. electrical insulation cables) and ability to mimic natural materials such as human skin (e.g. Barbie dolls).
PVC polymerization involves chemically adding thousands of vinyl chloride gas molecules together to produce a rigid solid material with a high molecular weight. Plasticizers or softeners are essential to increase the workability, flexibility and elongation of PVC and act by lubricating each polymer chain. Plasticization may be visualized as tossing cooked spaghetti (polymers) in olive oil to prevent the individual noodles sticking together. The more plasticizer, the more flexible the resulting PVC plastic. For example, electrical cables contain up to 30% plasticizer by weight, while fishing waders contain up to 50%. Phthalates â the chemicals used to soften, strengthen and improve the flexibility of plastics â have been widely used as plasticizers since the 1950s. However, after their identification as endocrine disruptors, their use today is frequently restricted and they are often substituted with alternatives.
Spacesuits developed for the Apollo program (1962-1972) were groundbreaking in their design, materials and construction. Although more than 50 years have passed since completion of the final Apollo mission, the spacesuits worn by Andreas Mogensen retain many of the original design features. See Figure 19. For example, the spacesuits and liquid cooling undergarments comprise at least ten different plastic types that protect the wearer and allow them to breathe, move unhindered, maintain a comfortable body temperature and carry out extra-vehicular activities using a Portable Life Support System. Liquid cooling undergarments comprise a tight-fitting fabric with a network of thin plasticized PVC tubing sewn onto the fabric. Circulating water in the tubing transports heat away from the astronautâs skin. The Portable Life Support System is a backpack that connects to the space suit via plasticized PVC hoses. They transport breathable oxygen to the wearer.
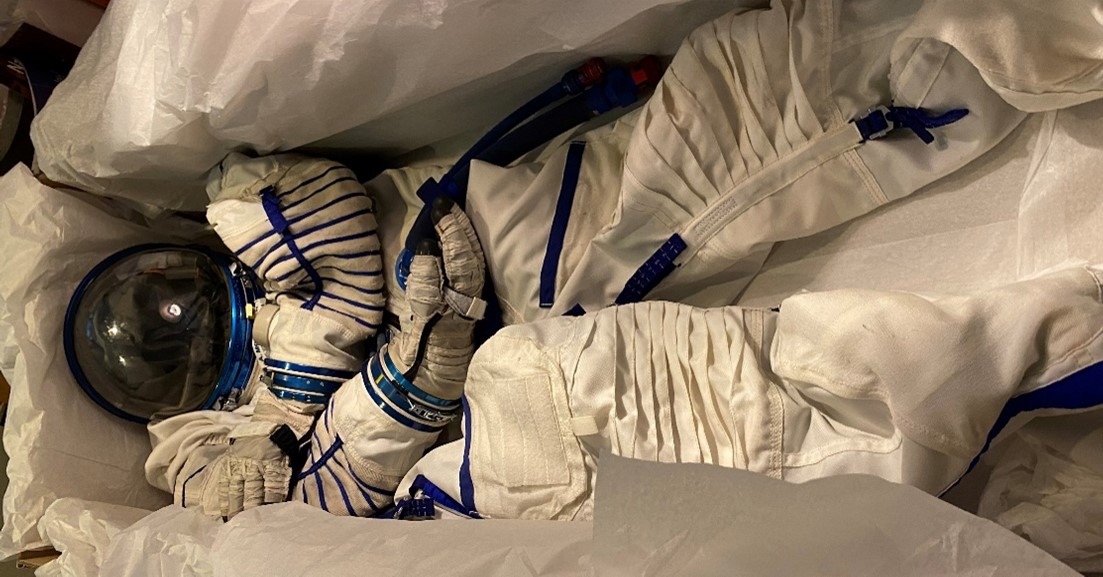
Figure 19. Spacesuit worn by Andreas Mogensen during a flight to the International Space Station in 2015, now in the collections of the National Museum of Denmark. The PVC hoses connecting to the Portable Life Support System can be seen supported by the right glove and crossing the body of the suit.
A 2000 study of the earlier spacesuits from the Apollo program undertaken by the National Air and Space Museum in Washington DC revealed that the plasticized PVC tubing in the liquid cooling undergarments as well as the hoses in the Portable Life Support System were stiff, had already changed colour (from transparent to dark brown), were tacky to the touch and their surfaces were obscured by white crystals. See Figure 20. Analyses suggested that the discolouration was due to oxidation of PVC polymer, the tackiness was caused by migration of phthalate plasticizer to surfaces and a subsequent chemical reaction with water produced white, phthalic acid crystals (Shashoua et al. 2002). To proactively combat such degradation, a preventive conservation strategy has been developed by the National Museum of Denmark to prolong the lifetime of plasticized PVC in Andreas Mogensenâs suit beyond the 30 years achieved by the Apollo Space programâs materials.
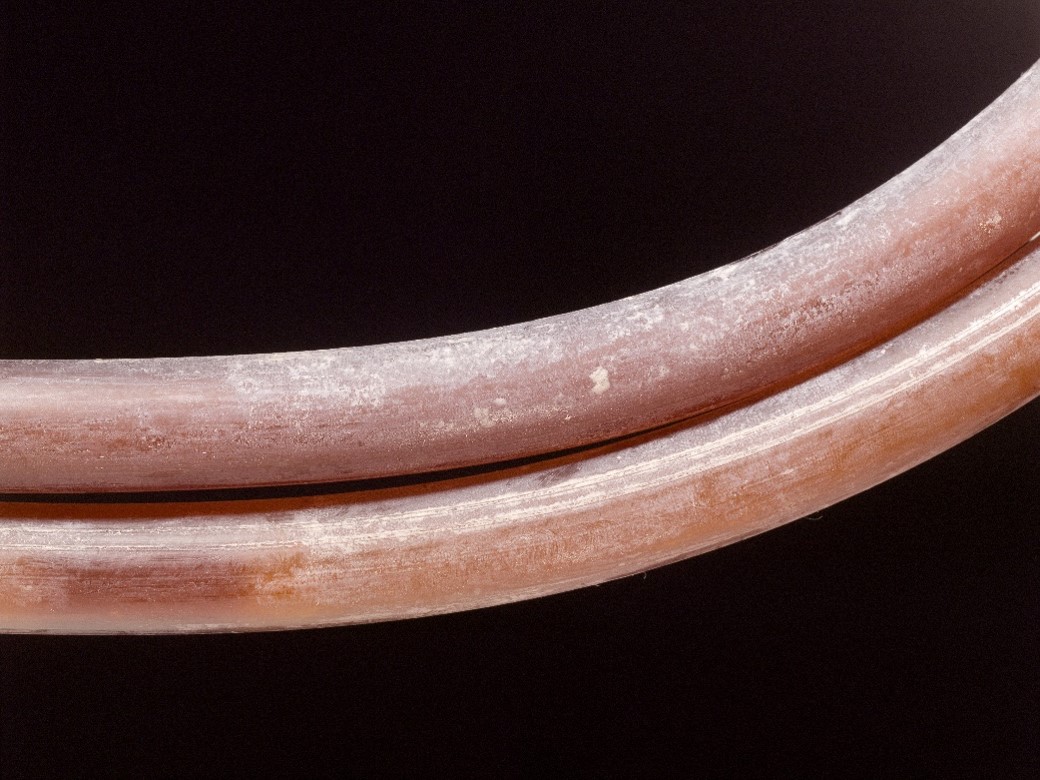
Figure 20. PVC tubing from the Apollo spacesuits became stiff, discoloured and developed white crystals at surfaces after 30 years in storage.
Conservation and archaeology need plastics!
Low density polyethylene (LDPE) was synthesized from ethylene gas by chance in 1933 and was used to insulate radar sets at the outbreak of World War II. Today it is the most produced polymer globally. Its success as a polymer is due to its long, branched chain structure that imparts high flexibility without the need for plasticizers. LDPE can withstand temperatures ranging from â50°C to 85°C. Because LDPE is highly resistant to water, water vapour, acids and bases but permeable to oxygen and carbon dioxide, it is used for bags, packaging films, geomembranes and containers for transporting and storing food and chemicals.
LDPEâs optical, thermal and chemical properties make it suitable for labelling, protecting, storing and treating archaeological artefacts and site samples including soils, geological materials and water. Resealable or ziplock bags have been commercially available since 1973 and evolved from the Danish inventor Borge Madsenâs "separable fastener" design, that replaced zipper teeth with interlocking plastic tracks (Macneal 2015). Unlike the paper envelopes and glass containers previously used to package artefacts and samples, enclosing artefacts and samples in resealable LDPE bags prior to storage in a refrigerator or freezer (at 4°C and -20°C, respectively) allows immediate access to the artefacts while also providing protection from condensation when removed from the cold. See Figure 21.
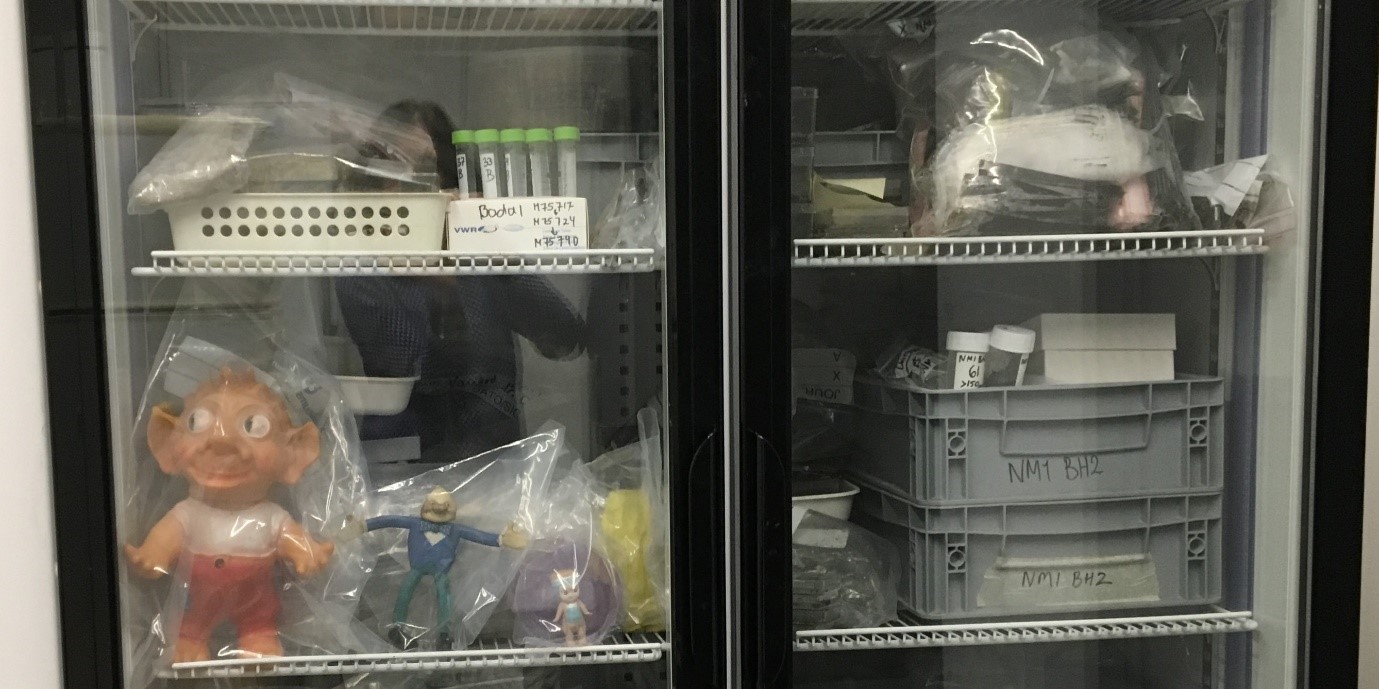
Figure 21. Museum objects and archaeological samples stored in a fridge are both accessible and protected by enclosing them in polyethylene bags.
Polyethylene sheets, bags and food containers are also used in terrestrial and marine environments to store samples and to preserve sites and remains or as part of in situ conservation. Much of the data informing on the time and pathways taken for plastics to degrade in natural environments are based on modelling studies, where samples are exposed to high intensities of light for short periods, and the changes that occur in their properties are recorded. From this, the data are extrapolated out to model the effects at the scale of years (Chamas et al. 2020). However, there can be a great deal of variability in the resulting models. For example, reported degradation times for plastic bags range from 10â1000 years.
A recent study of the degradation of plastics including polyethylene bags and containers in real time in coastal weather and marine environments over a three-year period suggested that LDPE degraded significantly faster when exposed to air, sunlight and precipitation than underwater or in sediments where the concentration of light and oxygen is lower (Shashoua et al. 2024). See Figure 22. In addition, black bags and containers showed greater resistance to oxidation than white and transparent examples.
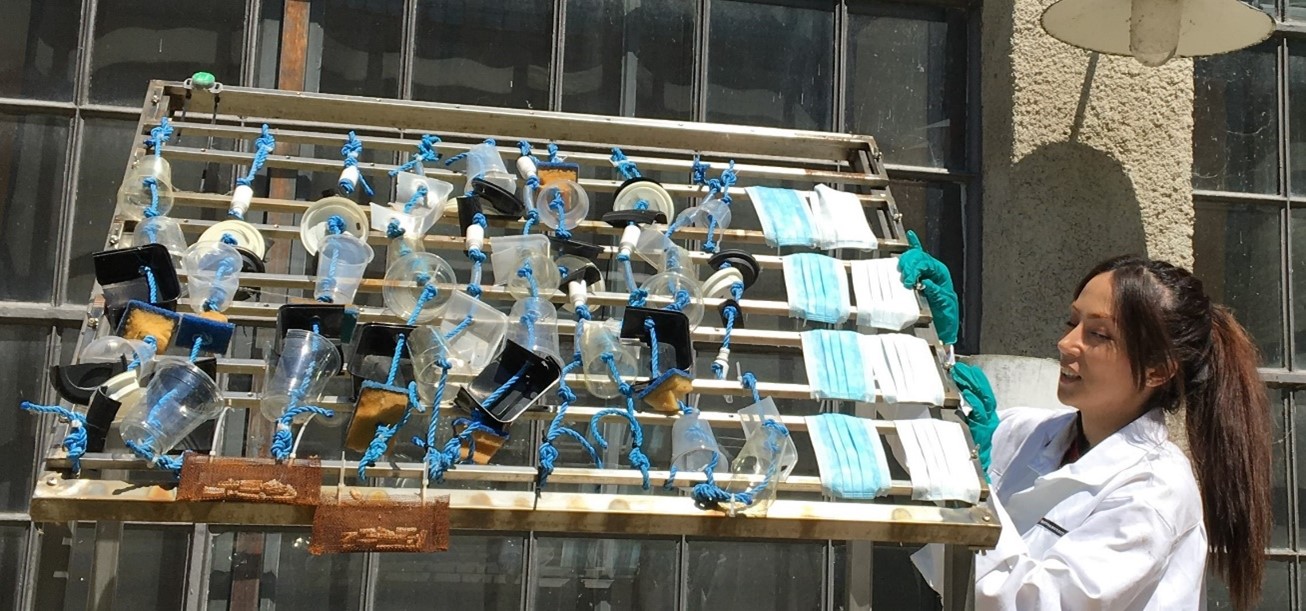
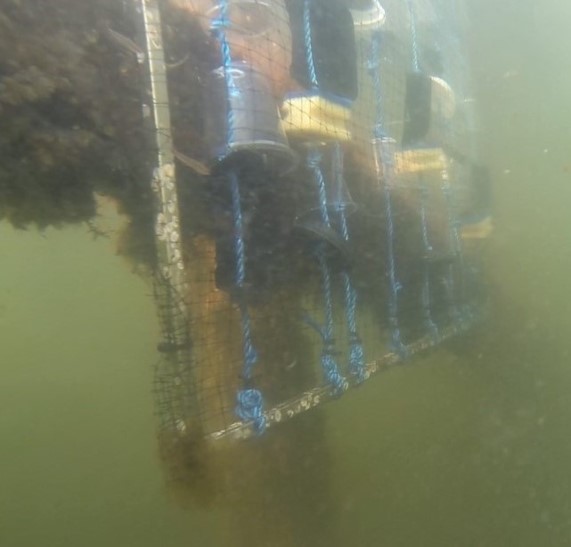
Figure 22. In a recent study by the National Museum of Denmark, plastic bags and containers were exposed in real time to weather (top image) and underwater (bottom image) for three years and their degradation pathways and rates measured.
Conclusion
Plastics have revolutionized our daily lives in the fields of technology, medicine, clothing, food preservation, sport, art and more. However, no organic materialsâincluding humans! âretain their original properties or functions forever. One major difference between plastics and other materials such as metals, ceramics and stone are their significantly shorter useable lifetimes. The challenges raised by the degradation of plastics in museums were recognized formally in 1991 largely inspired by the international conference âSaving the Twentieth Century: The Conservation of Modern Materialsâ. Concerns raised at the conference, and subsequent discussions and research resulted in the development of novel techniques to prolong the lifetimes of plastic artefacts and artworks (Grattan 1993). The formal identification of microplastics in the marine environment took place in 2004 and initiated a dramatic increase in research into the origins and impacts of these particles (Thompson et al. 2004).
More than thirty years after the degradation of plastics was recognized and acted upon in museums and twenty years after microplastics were first discovered on a beach in the UK, the first evidence of microplastic contamination in archaeological sediments was published (Rotchell et al. 2024). Almost simultaneously, the terms âwickedâ and âtoxicâ became associated with plastics and cultural heritage. However, to date, no scientific research has shown that microplastics have interacted chemically or physically with archaeological materials or damaged archaeological sites. Based on the three examples of different types of plastics discussed here, plastics have contributed much to our technological development. They continue to impact our collections and our professional practices in both positive and negative ways. While concerns regarding the roles and impacts of different types of plastics are valid, interesting and certainly worth continued scrutiny, I would argue that it is too soon for archaeology to simply cancel them.
Bibliography
British Archaeology. 2024. Small wins and wicked problems. British Archaeology. May June 2024, pp 34-39 https://www.archaeologyuk.org/resource/open-access-article-small-wins-and-wicked-problems.html accessed 20 December 2024.
Chamas, A., Moon, H., Zheng, J., Qiu, Y., Tabassum, T., Jang, J. H., Abu-Omar, M., Scott, S. L., & Suh, S.2020. Degradation Rates of Plastics in the Environment. ACS Sustainable Chemistry and Engineering. 8(9), pp.3494â3511. https://doi.org/10.1021/acssuschemeng.9b06635 accessed 20 December 2024.
Chen, Y., Awasthi, A. K., Wei, F., Tan, Q., & Li, J. 2021. Single-use plastics: Production, usage, disposal, and adverse impacts. Science of the Total Environment (Vol. 752). Elsevier B.V. https://doi.org/10.1016/j.scitotenv.2020.141772 accessed 20 December 2024.
Grattan, D.W.1993. Saving the Twentieth Century: The Conservation of Modern Materials. Ottawa: Canadian Conservation Institute. ISBN: 9780660578545.
Huo,Y., Dijkstra,A.F., Possell, M. & Singh,B. 2024.Mineralisation and priming effects of a biodegradable plastic mulch film in soils: Influence of soil type, temperature and plastic particle size. Soil Biology and Biochemistry. 189,1092570717. https://doi.org/10.1016/j.soilbio.2023.109257 accessed 20 December 2024.
Macneal, D.2015. The Surprisingly Complex Design of the Ziploc Bag. Wired. https://www.wired.com/2015/04/surprisingly-complex-design-ziploc-bag/ accessed 20 December 2024.
McNeill, I.C.1992.Fundamental aspects of polymer degradation. In N.S. Allen, M. Edge and C.V. Horie (eds.) Polymers in Conservation. London:Royal Society of Chemistry, pp.14-31.
Meade, R. K., McCormack, H., Clark, L. T., Sclater, S. G., Alexander, G. & Lamborn, L. 1905. Chemical Age. McCready Publishing Company. https://books.google.it/books?id=kwA3AQAAMAAJ&pg=PA288&redir_esc=y accessed 20 December 2024.
Plastics Europe. 2021. Plastics - the Facts 2021. https://plasticseurope.org/wp-content/uploads/2021/12/Plastics-the-Facts-2021-web-final.pdf accessed 20 December 2024.
Rotchell, J.M., Mendrik,F., Chapman, E., Flintoft,P., Panter,I., Gallio, G., McDonnell, C., Liddle, C.R., Jennings, D. & Schofield, J.2024. The contamination of in situ archaeological remains: A pilot analysis of microplastics in sediment samples using ÎŒFTIR. Science of The Total Environment. 914. https://doi.org/10.1016/j.scitotenv.2024.169941 accessed 20 December 2024.
Shashoua, Y., Schnell, U. & Young, L. 2002. Deterioration of plasticized PVC components in Apollo spacesuits. In T. van Oosten, Y.Shashoua and F.Waentig (eds.) Plastics in Art, History, Technology, Preservation. Munich:Siegl, ISBN: 3-935643-05-5. pp 69-79.
Shashoua, Y.,Peydaei, A., Mortensen, M.N., Kanstrup, A.B. &Gregory, D.J.2024. Physio-chemical degradation of single-use plastics in natural weather and marine environments. Environmental Pollution. 357. 124414. https://doi.org/10.1016/j.envpol.2024.124414 accessed 20 December 2024.
Stannett, V. 1951. Cellulose Acetate Plastics. New York:Chemical Publishing Co.
Thompson, R. C. , Olsen, Y., Mitchell, R.P., Davis, A., Rowland, S.J., John, A.W.G., McGonigle, D. & Russell, A.E. 2004. Lost at Sea: Where Is All the Plastic? Science 304, 5672, 838. DOI: 10.1126/science.1094559 accessed 20 December 2024.
Weiss, M., Haufe,J., Carus, M., BrandĂŁo,M., Bringezu, S., Hermann, B. & Patel, M.K. 2012. A Review of the Environmental Impacts of Biobased Materials. Journal of Industrial Ecology 16, s1, S169-S181. doi:10.1111/j.1530-9290.2012.00468.x accessed 20 December 2024.
Williams, S.R. 2002. Care of plastics: malignant plastics, WAAC newsletter (Western Association for Art Conservation). 24. 1. ISBN 10520066 pp.10-15.
Zhang, K., Hamidian, A. H., TubiÄ, A., Zhang, Y., Fang, J. K. H., Wu, C., & Lam, P. K. S. 2021. Understanding plastic degradation and microplastic formation in the environment: A review. Environmental Pollution. 274. https://doi.org/10.1016/j.envpol.2021.116554 accessed 20 December 2024.
Go back to top